When the video above starts, this wiggling, glowing blue blob seen under a new high-resolution microscope doesn’t look like much. But in just 26 seconds you and I can watch the blob’s tiny cells multiply, interact and organize into the first organ systems of a living mouse embryo.
The hollow crater forming on the left will give rise to the mouse’s stomach, pancreas and liver. The narrow white line stretching across the center of the image is the notochord, or an early backbone. And the contracting region on the far-left side of the image is the beginning of a heartbeat.
Researchers at the Howard Hughes Medical Institute’s Janelia Research Campus in Ashburn, Va., built a new microscope that can trace individual cells’ origins and movement in real-time, sketching a virtual map of how mammals develop in the womb.
The results, published on Thursday in the journal Cell, could have critical applications for understanding how organ systems form in humans as well, potentially assisting treatments of birth defects.
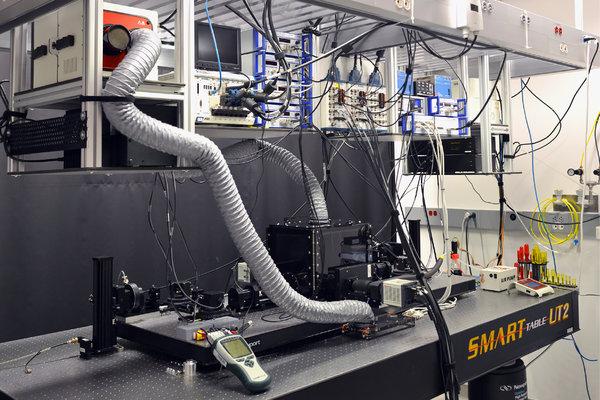
CreditKatie McDole et al.
“We have been previously limited to snapshots in time, like reading random pages torn out of a book,” said Kate McDole, a developmental biologist and one of the study’s co-authors. “But how are the organs actually formed? What’s the timing, the tissues, the dynamics that are involved? You can only study that by looking at it live.”
“This work fills in all the gaps and tells a complete story,” Dr. McDole added.
[Like the Science Times page on Facebook. | Sign up for the Science Times newsletter.]
The research team, led by Philipp Keller, a physicist and biologist, set out to overcome the limitations of confocal microscopy, a traditional imaging method that casts light through an entire specimen for extended periods of time.
That method was too harsh for the delicate embryonic cells of a mouse. “You’d basically be microwaving it,” Dr. McDole said.
The new microscope — a clear, acrylic cube with a central chamber — has two cameras and two razor-thin sheets of light illuminating only small portions of the specimen as it bobs in a nutrient-rich fluid. Every few milliseconds, algorithms take into account the changing position of the embryo, the angle at which the light sheets are striking it, and the best direction from which to capture a clear image.
The team collected almost one million frames of each live embryo and compiled them, Dr. Keller said.
Using a cell-tracking program, they followed each individual cell through a critical 48-hour window — from six-and-a-half to eight-and-a-half days after fertilization — during which the embryo grows to about the length of a sesame seed and its vital organs begin to form.
Below, embryonic stem cells fold together to form a mouse’s heart. Each white dot is an individual cell, migrating to create an atrium, the endocardial membrane (the emerging “X” shape) and finally a heartbeat.
Published OnCreditCreditImage by K. McDole et al./Cell 2018/Janelia Research Campus
The team also created a cell division detector to track when, where and which cells divide, as well as a tool that portrays a virtual “average” mouse embryo by aligning four of the samples. (They named it TARDIS after the vehicle that transports the Doctor and his or her companions through time and space on “Doctor Who.”)
A demonstration of the TARDIS tool.Published OnCreditCreditImage by K. McDole et al./Cell 2018/Janelia Research Campus
Scientists are not permitted to experiment with human embryos. But by visualizing how organs form in mice, this research could help doctors investigate developmental issues inside the human womb.
A birth defect called spina bifida, for example, occurs when a portion of a fetus’s neural tube fails to seal properly. The footage below — captured from the underside, with the head on the left and the tail on the right — shows the neural tube closing as it should.
Watch as the two prominent lines stretching across the embryo join together, with somites (later, ribs) forming on either side.
A neural tube in a mouse’s fetus closes properly.Published OnCreditCreditImage by K. McDole et al./Cell 2018/Janelia Research Campus
The researchers also believe that, in time, studying the blueprints in motion could inform scientists’ endeavors to grow or regenerate organs in a lab.
“Put a bunch of stem cells in a dish, dump in chemicals and create things that look like cardiac cells. But that only gives you the building blocks,” Dr. McDole said. “You can’t take the pieces of a house and throw them in a pile. What we’re doing here is really trying to understand how the roof goes on.”
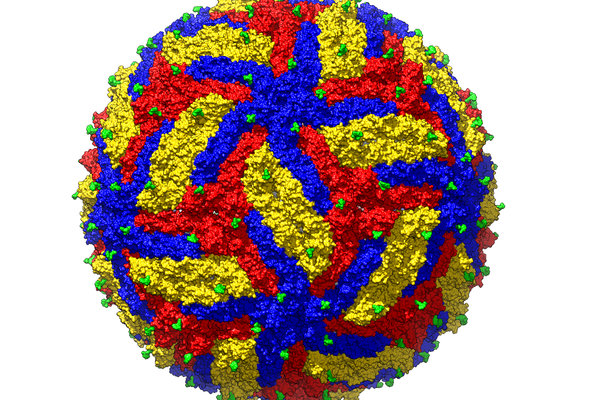
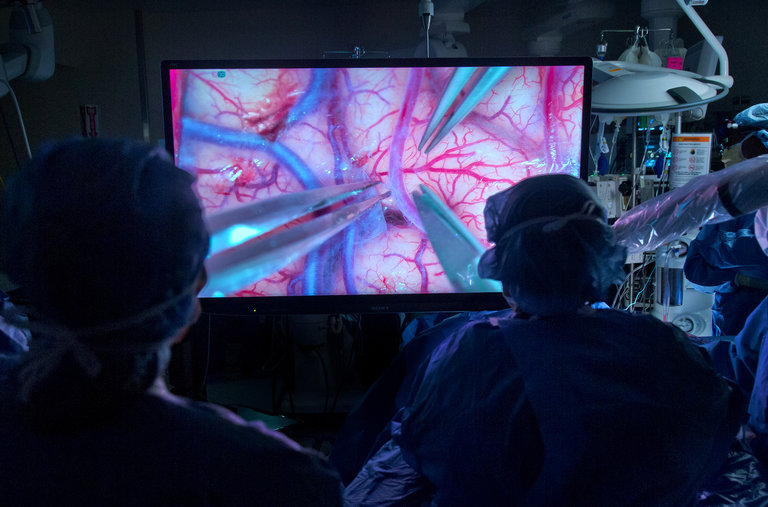
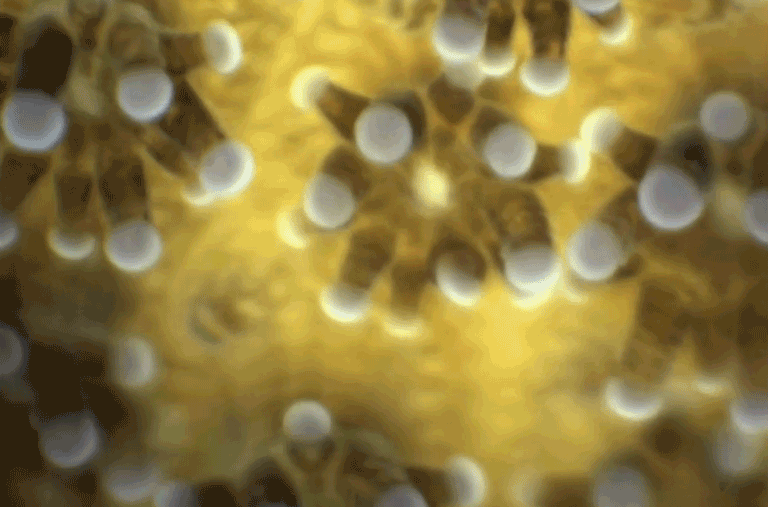