SAN DIEGO — Two hundred and fifty miles over Alysson Muotri’s head, a thousand tiny spheres of brain cells were sailing through space.
The clusters, called brain organoids, had been grown a few weeks earlier in the biologist’s lab here at the University of California, San Diego. He and his colleagues altered human skin cells into stem cells, then coaxed them to develop as brain cells do in an embryo.
The organoids grew into balls about the size of a pinhead, each containing hundreds of thousands of cells in a variety of types, each type producing the same chemicals and electrical signals as those cells do in our own brains. In July, NASA packed the organoids aboard a rocket and sent them to the International Space Station to see how they develop in zero gravity.
Now the organoids were stowed inside a metal box, fed by bags of nutritious broth. “I think they are replicating like crazy at this stage, and so we’re going to have bigger organoids,” Dr. Muotri said in a recent interview in his office overlooking the Pacific.
What, exactly, are they growing into? That’s a question that has scientists and philosophers alike scratching their heads.
On Thursday, Dr. Muotri and his colleagues reported that they have recorded simple brain waves in these organoids. In mature human brains, such waves are produced by widespread networks of neurons firing in synchrony. Particular wave patterns are linked to particular forms of brain activity, like retrieving memories and dreaming.
As the organoids mature, the researchers also found, the waves change in ways that resemble the changes in the developing brains of premature babies.
“It’s pretty amazing,” said Giorgia Quadrato, a neurobiologist at the University of Southern California who was not involved in the new study. “No one really knew if that was possible.”
But Dr. Quadrato stressed it was important not to read too much into the parallels. What she, Dr. Muotri and other brain organoid experts build are clusters of replicating brain cells, not actual brains.
“People will say, ‘Ah, these are like the brains of preterm infants,’” she said. “No, they are not.”
It’s been only six years since scientists created the first brain organoid from human skin cells. Now they’re being grown in laboratories around the world, offering scientists a new window onto the earliest stages of human brain development.
Here at U.C.S.D., researchers are using them to recreate, in miniature, inherited brain disorders and brain infections. They are also trying to grow bigger, more complex brain organoids. In one recent experiment, scientists linked a brain organoid and a spider-shaped robot, so that the two could exchange signals.
With Thursday’s report, published in the journal Cell Stem Cell, the question of what brain organoids might become is gaining more urgency.
“There are some of my colleagues who say, ‘No, these things will never be conscious,’” said Dr. Muotri. “Now I’m not so sure.”
Even if scientists someday produce only minimally self-aware organoids, that could represent a serious ethical concern, said Christof Koch, the chief scientist and president of the Allen Brain Institute in Seattle.
“The closer we come to his goal, the more likely we will get a brain that is capable of sentience and of feeling pain, agony and distress,” Dr. Koch said.
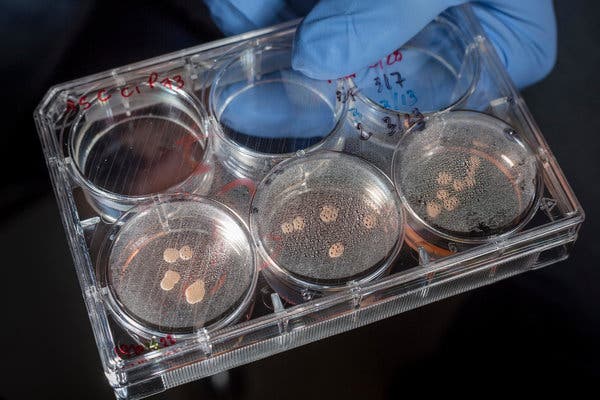
‘Failing miserably’
Few things in biology are harder to study than the development of the human brain. Scientists have largely contented themselves with indirect clues from studies of animals, like mice and monkeys.
But the human brain is so distinctive that it’s hard to make the evolutionary leap from other species. As a result, researchers have a disappointing track record for treating brain-based disorders such as autism or schizophrenia.
“We are failing miserably,” Dr. Muotri said. “We can cure animals of some diseases, but it’s not translatable.”
In 2006 Shinya Yamanaka, a biologist at Kyoto University in Japan, opened a new way to study human brains. He found a cocktail of four proteins that can turn ordinary skin cells into stem cells, which then have the potential to turn into neurons, muscles or blood cells.
Building on that advance, other researchers learned to get stem cells to grow like miniature organs in a dish. And in 2013, a team of researchers in Austria succeeded in producing small, short-lived brain organoids for the first time.
[Like the Science Times page on Facebook. | Sign up for the Science Times newsletter.]
Until then, Dr. Muotri had studied neurons derived from people with autism. He quickly taught himself how to turn stem cells into brain organoids instead.
“The most incredible thing is that they build themselves,” he said. Primed with just the right conditions, the organoids take over their own development.
Today, Cleber Trujillo, a project scientist, oversees the growth of thousands of organoids in a tissue culture room in Dr. Muotri’s lab. “This is where we spend half our day,” he said, gesturing to the banks of refrigerators, incubators and microscopes.
Brain organoids demand so much labor because growing them is more like making a soufflé than running a chemistry experiment. Scientists have to continually replace the broth in which the cells grow and keep a careful eye on the cells themselves.
“We need to guide them,” said Dr. Trujillo. “Otherwise, they become other stuff.”
If all goes according to plan, the cells turn into brain organoids. They become blobs, inside of which tunnels form. So-called progenitor cells surround the tunnels and sprout cables. Other cells crawl down the cables and form successive rings.
They match the cells in our cortex in many respects. Their surface even folds in on itself.
Dr. Trujillo pulled out a translucent muffin tray and raised it above his head. The lights overhead illuminated hundreds of tiny pale spheres. At two months, the cells inside each organoid held it together with a network of sticky branches.
“They like to stay in touch with each other,” Dr. Trujillo said fondly.
Dr. Muotri and his colleagues use brain organoids to run experiments on a host of diseases.
They have infected organoids with Zika virus, for instance, to better understand how it causes severe brain damage in babies. Last year, the researchers discovered that a drug called sofosbuvir, already approved for hepatitis, shields brain organoids from infection.
Dr. David Baud, a Zika expert at the University of Lausanne in Switzerland, who was not involved in the study, called it a promising discovery — one that would be hard to make studying mice or individual neurons. “Organoids offer the best alternative,” he said.
Fabio Papes, a neuroscientist at University of Campinas in Brazil, feels the same way about organoids when it comes to studying hereditary brain disorders. Dr. Papes is collaborating with Dr. Muotri to study a disease called Pitt-Hopkins syndrome.
It’s a rare condition that leaves children unable to speak and suffering regular seizures. To understand how certain mutations cause the disease, Dr. Papes is growing organoids from skin cells donated from patients.
“For this particular disease, mice are no good,” he said. “And you obviously cannot open the child’s head to know what’s going on. We can go this way, or not go at all.”
A critical mass
In 2016 Priscilla Negraes, then a project scientist in Dr. Muotri’s lab, began eavesdropping on organoids. She figured out how to stick them to the bottom of a well that was lined with 64 electrodes. When a neuron in the organoids fired, one of the electrodes would light up.
The organoids turned out to be surprisingly noisy — and with each passing week, they got noisier. Then she noticed that patterns were emerging.
Most of the neurons would suddenly start firing in a synchronized burst — a pattern that looked remarkably like brain waves.
Dr. Negraes and her colleagues began working with brain wave experts, and they found some similarities between the organoids and the brains of premature infants. The babies and the organoids both produced bursts of synchronized activity, followed by quiet lulls.
As the organoids grew, the researchers found, the lulls got shorter. That, too, is a feature of premature baby brains as they mature.
What makes the similarity so remarkable is that organoids and baby brains are so different, said Richard Gao, a graduate student at U.C.S.D. and a co-author of the new study.
“I don’t think you need all the billions of neurons in the brain to produce these patterns,” Mr. Gao said. “Once you pass a critical mass, it’s able to do that.”
To learn more from brain organoids, researchers want to make them bigger, more complex, and more durable. Immune cells, surprisingly, may make that possible.
Immune cells called microglia don’t just fight pathogens. In the developing brain, they sculpt neuron branches, helping them to mature. Researchers in Dr. Muotri’s lab have coaxed microglia to crawl inside brain organoids. Now the scientists are tracking the organoids’ activity as they develop.
“I’m thinking, with microglia it might be better,” said Gabriela Goldberg, a graduate student on the project.
Real brains need connections to the outside world to mature properly. In 2017, Dr. Quadrato and her colleagues grew a brain organoid that included cells from the retina, making it sensitive to light.
Dr. Muotri and his colleagues are testing out different ways to stimulate brain organoids in order to make them develop more complex neural networks. In one experiment, they’ve linked organoids to a small spider-shaped robot. A computer translates an organoid’s electrical activity into instructions for moving the robot’s legs.
As the robot moves, it uses sensors to detect when it gets close to a wall. The computer relays those signals back to the organoid in the form of electrical pulses.
Dr. Muotri can’t say yet whether these experiments will affect how brain organoids develop. The efforts may fail — or they may produce increasingly sophisticated brain mimics that we don’t fully understand.
He urges his fellow scientists to think carefully about what they may inadvertently create.
“What if you add neurons that sense pain?” asked Dr. Muotri. “Or what if we start recording memories in these organoids?”
But Jeantine Lunshof, a bioethicist at Harvard University, believes it’s too early to make judgments about what we should and shouldn’t do with brain organoids.
“In order to say what you should do with it, you first have to say, ‘What is it?’” she said. “We’re making things that were not known 10 years ago. They were not in the catalog of philosophers.”
At this stage of research, Dr. Lunshof said, the most important thing is to avoid mistaking today’s brain organoids for true human brains — let alone people. “They’re in a completely different category,” she argued.
For now, these debates matter to a few scientists: the master chefs who can reliably make enough brain organoids to run experiments. But Dr. Muotri and Dr. Trujillo hope to automate the process, so that other scientists can make lots of cheap, high-quality brain organoids.
“That’s our concept — plug and play,” said Dr. Muotri. “We want to make farms of these organoids.”
The organoids sent to space may help make that concept a reality. The box in which they were housed is a rough prototype of a device that someday might produce organoids without human intervention.
The astronauts aboard the space station simply installed the box, turned on the power, and let it run on its own.
On a recent morning, Dr. Muotri wanted to check on his space organoids. Cameras inside the box snap pictures every half-hour, but all the pictures Dr. Muotri had seen were obscured by unexpected air bubbles.
Now, to his delight, the bubbles were gone from the latest image. On his computer monitor, he saw a half-dozen gray spheres floating on a beige background.
“They’re rounded, and they more or less have the same size,” he said. “You don’t see them fusing or clustering together. So this is all good news.”
If all this work does eventually lead to mass-produced brain organoids, Dr. Muotri won’t mind if his artisanal organoid-growing skills become obsolete.
“I think we can use our brains for something more noble,” he said.